Basics of NMR
Experimental settings
Acquisition: Optimization of instrumentation and experimental parameters
- To obtain high-quality NMR data, it is essential to properly select and optimize a number of technical aspects:
- Sampling
- Selection of the measuring head depending on the signal to be recorded; matching and adaptation of the selected measuring head.
- Field-frequency “Lock” procedure to ensure temporal stability of B0 during acquisitions
- Adjustment of the magnetic field homogeneity on the sample studied: “Shim”
- Time optimization of the selected NMR sequence (AQ, D1) and optimization of spectral parameters (TD, SW, O1)
- Calibration of the radio frequency pulses used during acquisition
- Once the instrument settings and the experimental parameters are optimized, the acquisition is carried out in the best conditions. The resulting signal can be processed: it is necessary to adjust the data processing parameters of the acquired NMR signal (zero filling, apodization, phasing if possible)
Sampling
Depending on the physical state of the solute and the NMR information we wish to collect, it is necessary to adjust the sampling. Indeed, the dynamics of the solute are not the same in a viscous or non-viscous solvent. Also, the chemical exchange rates are different in a protic or aprotic solvent.
When the sample is liquid or liquid crystal, the appropriate container is usually a 20 cm long tube with a 5 mm standard outer diameter (o.d). The choice of tube diameter can vary from 1 to 15 mm depending on the amount of solute available, bearing in mind that the volume of analysis is fixed, and the type of nucleus excited. The quality of the tube (glass quality, parallelism and thickness of the tube walls) must be adapted to the amplitude of the magnetic field of the magnet so as not to be the limiting element of the homogeneity adjustment procedure. The resistance to high temperature and pressure fluctuations should also be considered if necessary. It is recommended not to scratch the walls of the tube nor to heat it during the cleaning phase: a suitable device exists for cleaning. The rotors supporting the tubes must also be adapted according to the temperature (ceramic for high and low temperatures, or PTFE in routine).
Zircon rotors rather than glass tubes are used for solid samples.
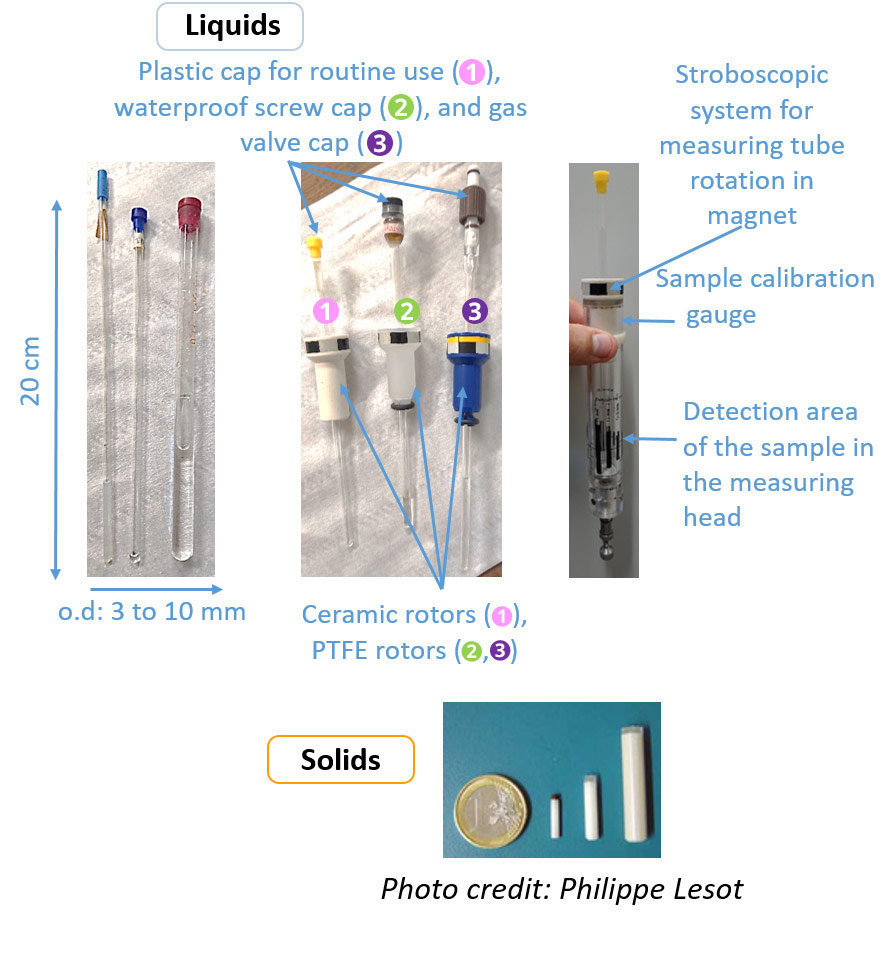
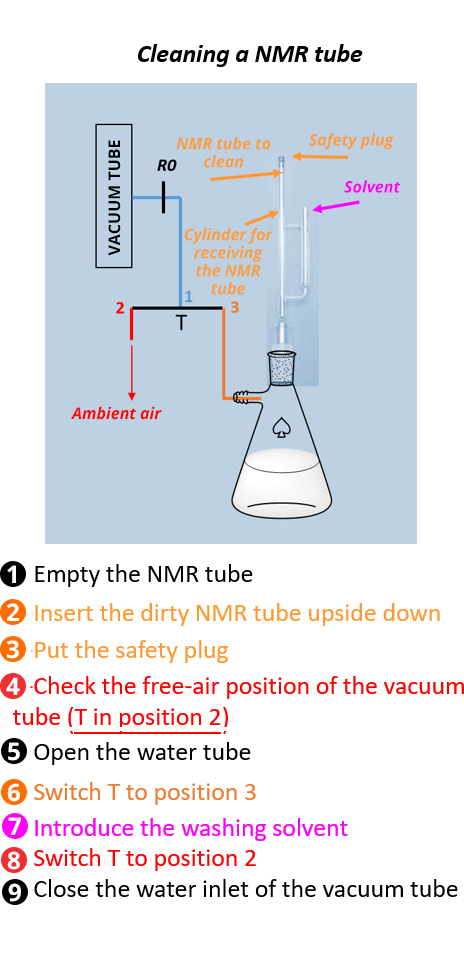
Matching and adaptation of a NMR measuring head
- To optimize the transmission-reception of a given signal, the {L, C1, C2} circuit must be tuned in frequency to ν ≈ νr : "Tuning" is done by modifying the value of C2. It must be adapted in impedance too, by changing the value of C1, so that the circuit is purely resistive: “Matching”.
- To change the value of a capacitance, simply change the spacing of the two plates that make up the capacitor. Thumbwheels or bars defined as "T" and "M" are positioned on the measuring probe for these "Tuning" and "Matching" steps.
- These steps must be repeated as soon as a new sample is introduced or whenever experimental quantities, such as temperature, are changed.
In the "Tuning and matching" window of the probe, the x-axis allows you to adjust the frequency (“Tuning”), and the y-axis is used to adjust the impedance ("matching"). The response curve of the RLC response circuit of the transmit-receive coil of the nucleus under consideration is presented in grey.
- “Tuning” setting: The minimum of this curve must coincide on the x-axis with the Larmor frequency of the considered nucleus. This frequency is symbolized by the vertical pink line. This is to favor good frequency tuning conditions, regardless of its position on the y-axis.
- “Matching” setting: The minimum of this curve must coincide with a zero value on the y-axis in order to favor good impedance adjustment conditions, regardless of its position on the x-axis.
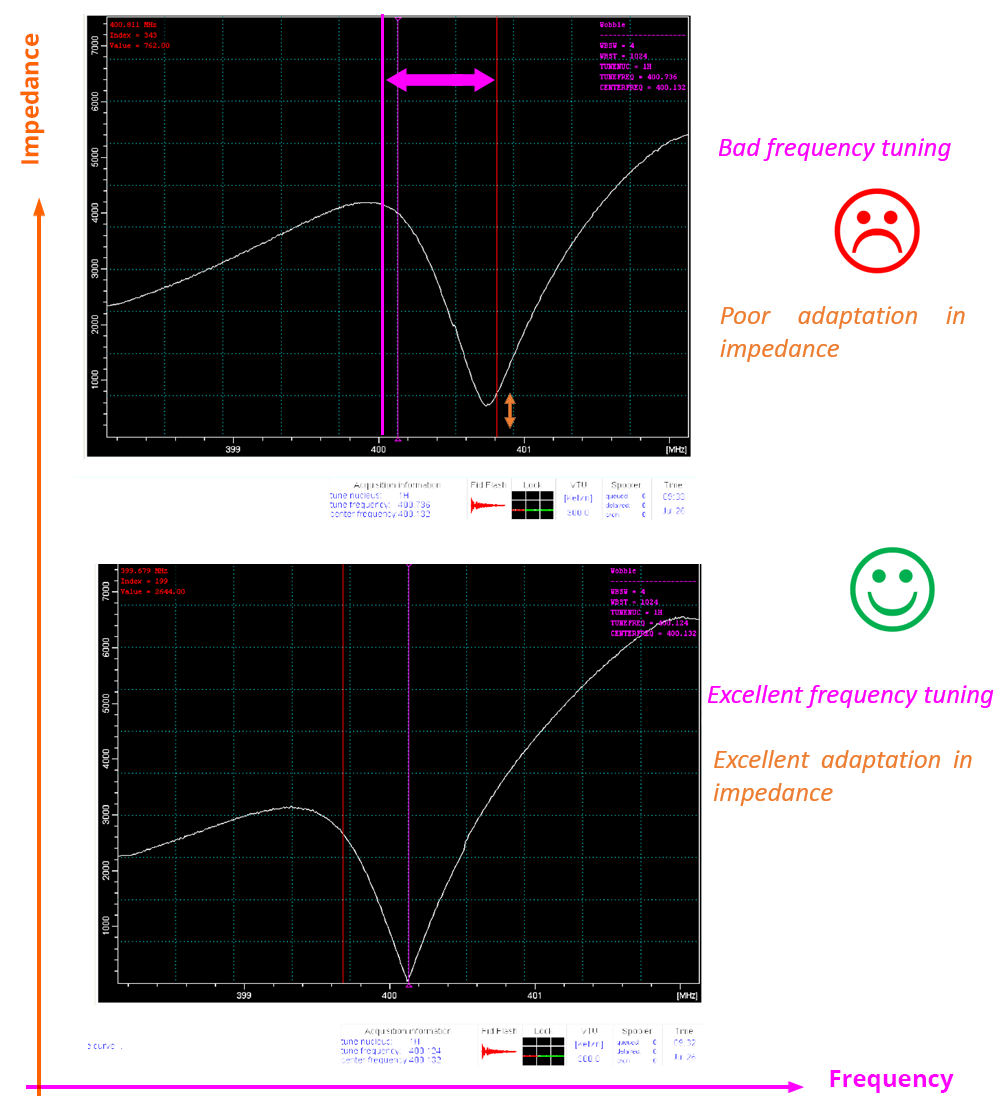
Temporal stability of B0 field: field-frequency lock or “lock” signal
In order to ensure the temporal stability of the B0 magnetic field, an electronic control loop (tuned to the frequency of a spin other than the one under study) is installed on the spectrometer. Usually, the "lock" circuit is tuned to the deuterium frequency. This requires the introduction of deuterium nuclei into the sample, generally using a deuterated sampling solvent that is chemically compatible with the compound of interest ("internal lock"). If it is not possible to pollute the sample, an "external lock" is preferred using two capillary NMR tubes, for example (one tube containing the solution of the compound of interest in a suitable solvent, the other containing the compound useful for the "lock" procedure).
If the B0 magnetic field has varied between two experimental points, the resonance frequency of the spy nucleus used for the “lock” will have varied like that of the excited nucleus. It will be reset to its nominal value by the electronic control loop before acquiring the signal from the excited nucleus.
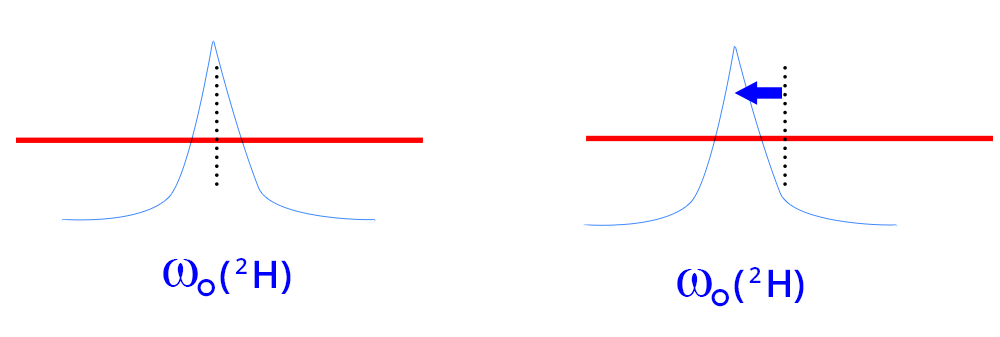
Homogeneity of the B0 field for all nuclei of the sample: “shims” procedure
The NMR spectrometer is equipped with a magnetic field magnet that is perfectly homogeneous throughout the acquisition area. To achieve this, a set of "cold shim coils" is installed in the superconducting magnet. These coils are inaccessible to the user.
When an NMR sample is introduced into the magnet, the quality of the NMR tube, as well as the nature of the sample components (solute, solvent, impurities, etc.) locally affect the parallelism of the B0 magnetic field lines. In order to compensate for these local inhomogeneities, the magnet is equipped with a concentric "shims barrel”. It contains a set of "ambient temperature shim coils" whose electrical intensity can be adjusted by the user. Thus, locally, small magnetic fields generated by these "shims" (and controlled by the user) will make it possible to re-obtain as-close-to-perfect-as-possible homogeneity of magnetic field, in the presence of the sample introduced into the magnet.
In general, the higher the intensity of the magnetic field, the more difficult it is to obtain perfect magnetic field homogeneity. However, there are now more or less efficient automated procedures to carry out the "shimming" step. Some map the magnetic field lines before and after sample introduction to achieve the desired result (“Topshim”, “gradshim”).
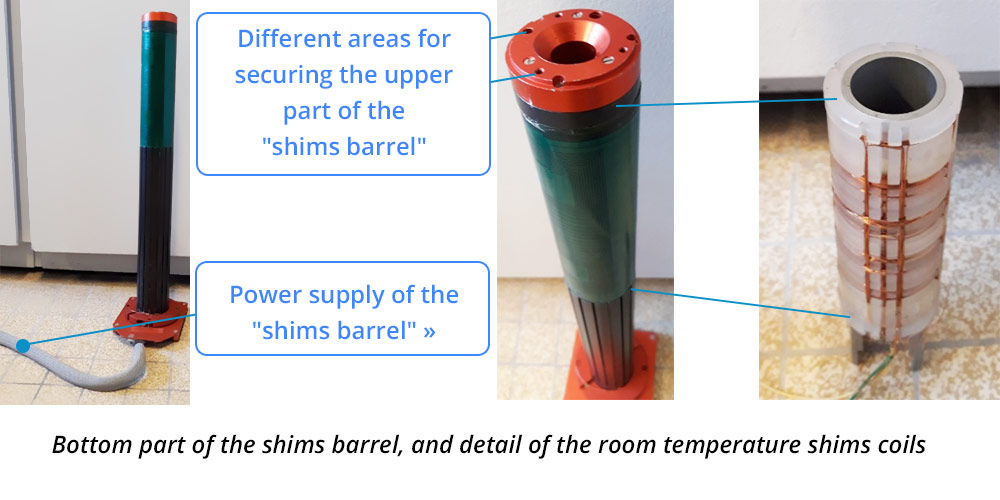
NMR spectral accumulation: minimum repetition time
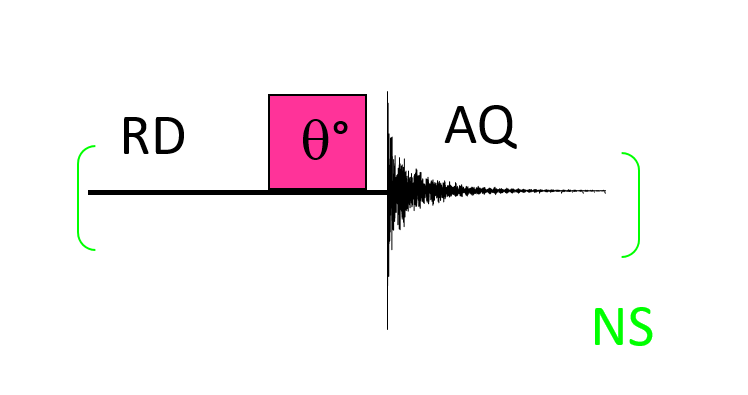
In order to be quantitative, all spin systems must have relaxed before they can move on to a new signal accumulation. The maximum signal is obtained for a pulse angle of 90° but requires waiting at least 5 times the T1 value of the slowest spin to relax, which can be time-consuming and incompatible with duration of the experiment. It is therefore necessary to find a good compromise between: 1-The waiting time between each accumulation of the signal, 2-The number NS of accumulations for a S/N ratio compatible with good quality integral measurements, and 3- The pulse angle applied, as shown in the following figure.
- Ernst's Angle θErnst (Nobel Prize in Chemistry 1991) is the best compromise between a given T1 value and the AQ + RD waiting time for a given accumulation.
- Therefore θErnst is such that
- Example: for T1 values of 3s, Ernst's angle equals 68°
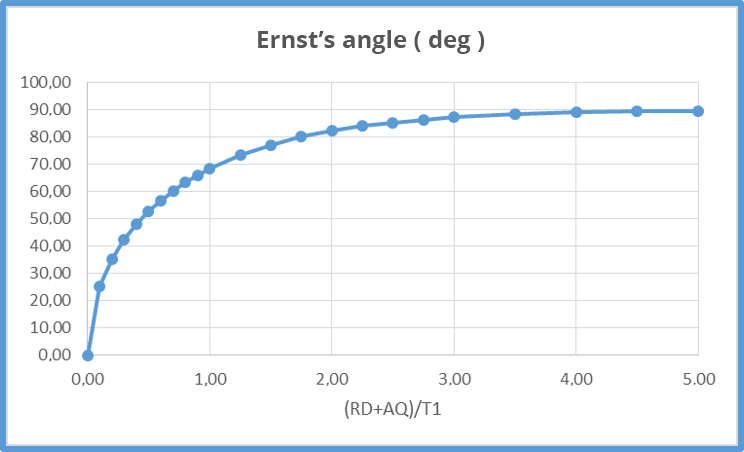
Digital acquisition and digital Fourier transform
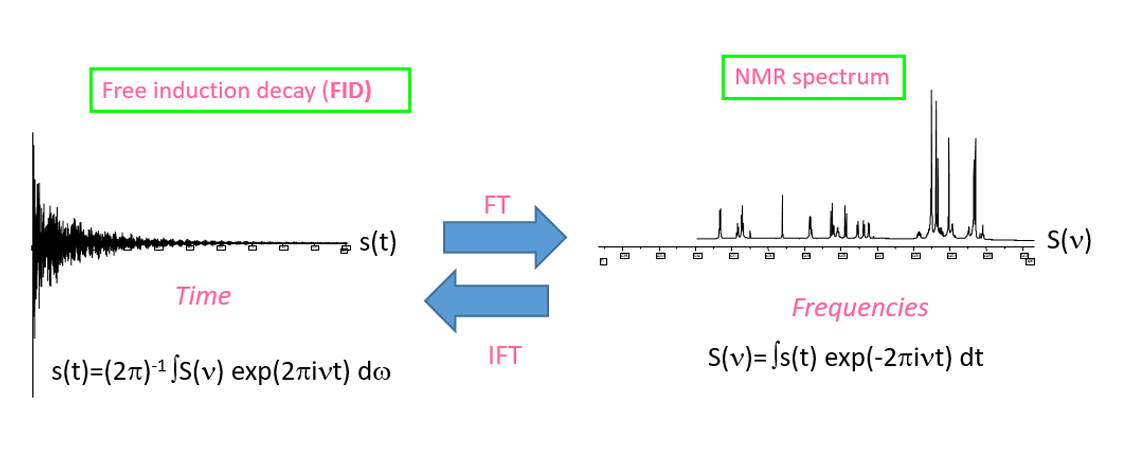
The NMR signal is analog since it is the response signal of the sample disturbed by a polychromatic radio frequency wave. For ease of processing, this analog signal is usually digitized using an analog-to-digital converter (ADC). The sampling of the analog signal into a digital signal is only accurate if it follows the Shannon-Nyquist theorem. Thus, the sampling frequency of the analog signal must be at least double the highest resonance frequency of the spin system studied.
In practice, AQ is empirically defined so that the FID reaches a (quasi)-null S/N ratio: AQ depends only on NMR interactions that govern the relaxation of the spin system. This FID is sampled by TD (Time Domain) points in order to respect the Shannon-Nyquist theorem. RD is set according to the chosen Ernst angle (PW), as well as NS having estimated the limit value of T1. After Fourier transformation, the NMR spectrum obtained has a spectral width SW centered on the value O1. SW and O1 must be empirically optimized considering the chemical functions present on the molecule or spin system studied.
The Cooley-Tuckey algorithm for fast Fourier transform (FFT) is only used when the number of points involved in the transformation is a power of 2. This condition generally implies carrying out the FFT not on TD points but on SI (Size) points=2N >TD
Calibration of a radio frequency pulse
In a mono- or multi-pulse NMR experiment, it is necessary to fully control and characterize the excitation of the nuclei studied.
In some multi-pulse sequences, the values of the tilt angles associated with the excitation pulses are defined well enough to achieve the desired result.
Even in the single-pulse sequence, the number of signal accumulations intimately depends on the chosen pulse angle, based on the relaxation properties of the spin system studied.
In any case, it is essential to calibrate the pulses used.
A radio frequency pulse corresponds to a certain electrical energy (characterized by its electrical power or its attenuation in dB) applied to the system for a duration PW. The usual pulses are polychromatic. They will consistently excite all the resonance frequencies of the spin system simultaneously, so that all nuclei begin to relax concomitantly. The calibration of these "broadband" pulses usually requires the electrical power to be fixed and PW to be varied.
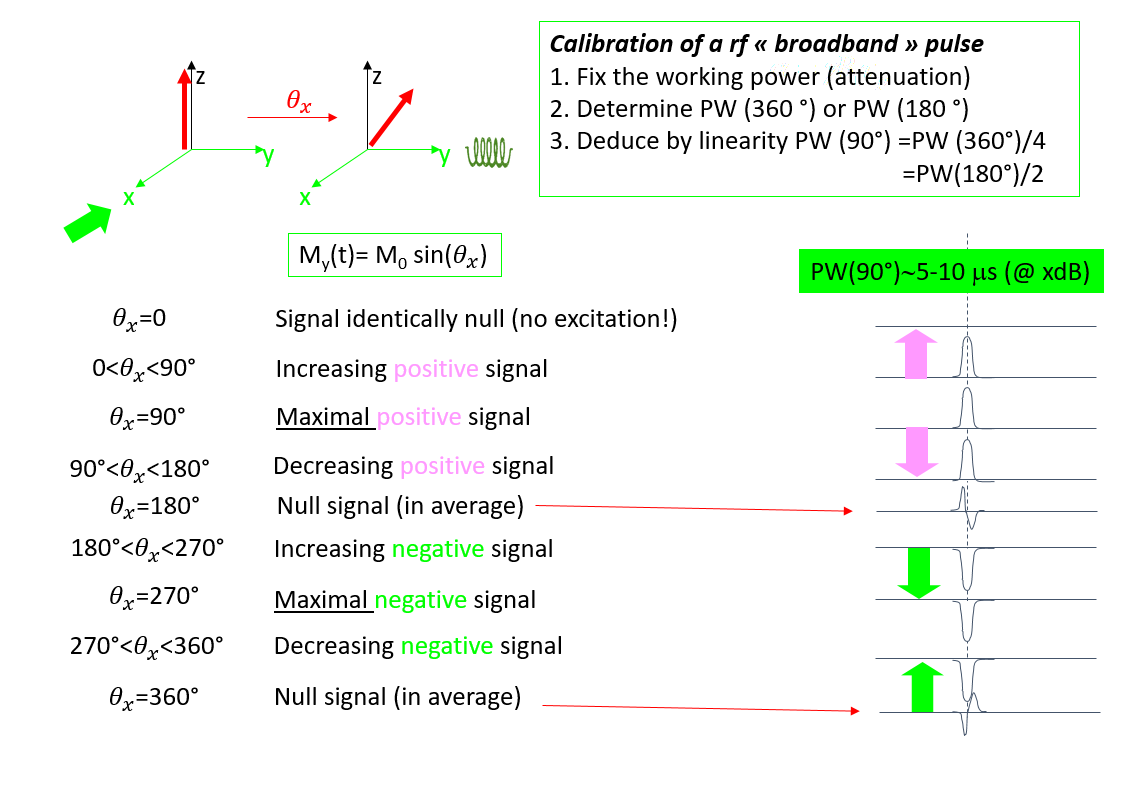
When calibrating the "band-selective" or selective pulses, the spectral window we wish to excite is fixed, which defines the pulse duration PW. Calibration then consists of defining the electrical power that corresponds to the desired tilting angle.